Keywords
|
Non-destructive testing, Magnetic flux leakage, Coercivity, Magnetic behavior. |
INTRODUCTION
|
Magnetic nano fluid are colloidal suspensions of superparamagnetic particles in a suitable carrier fluid. Magnetic nanofluids have attracted enormous interest in recent years because of their field driven self-assembly and tunable properties. The unique field driven properties of magnetic nanofluids have been exploited for several interesting applications such as electronics, cooling, sealant, dampers, optical filter, defect and ion sensors etc. Using the magnetically tunable optical properties of nano-emulsions, we have developed an optical probe for defect detection in ferromagnetic materials. In this work, we report a methodology to image defects in ferromagnetic materials and components using magnetic nano-fluids and the defect feature extraction and its quantification using image enhancing methods |
Magnetic flux leakage is one of the most commonly used electromagnetic nondestructive techniques (NDT). This technique has been extensively used in metal industries, to locate and asses the discontinuities such as fatigue, cracks, corrosion, erosion, non-metallic inclusion and abrasive wear in ferro-magnetic structures. MFL technique is found to have a high degree of certainty in the detection of defects with better sensitivity. The partial ferrite structure formed in this material, due to their imperfect heat treatments and mechanical stress are often wrongly diagnosed as a crack and sometimes real cracks are misjudged as ferrite, which can lead to catastrophic failures of engineering components. Lack of magnetization and over saturation of materials can leads to a poor flux leakage signal and overlap of signals with large background. For better visualization of the defects, the excitation flux should be sufficient to penetrate the defect depth and homogeneous throughout the specimen. The lift off distance of the sensor should be adjusted such that the defect signal is strong enough to detect distinctly from the background noise. These problems associated with conventional MFL techniques warrant the need to have more reliable, simple, cost effective and sensitive techniques for detection of leakage magnetic flux in ferromagnetic components and structures. |
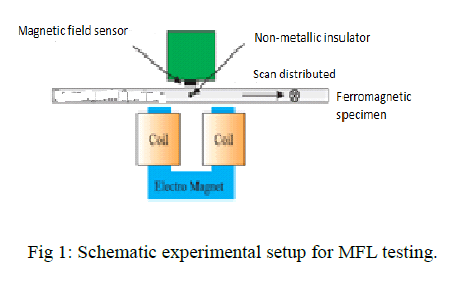 |
II. MATERIALS AND METHODS
|
Co-Precipitation is the incorporation of trace element into mineral structure during solid solution formation and recrystallization of minerals. This Process will reduce the mobility and toxicity of toxic trace elements that are incorporated in the mineral. |
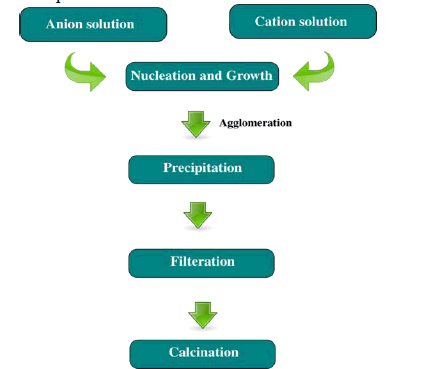 |
2.1 Synthesis of Fe2O3 nano particle
|
Iron ore tailings were weighed (100 g), and mixed with 37.5wt% hydrochloric acid (HCl). Then pickling was filtered out and collected. An appropriate amount of hydrogen peroxide (H2O2) was added to the filtrate so that all iron could exist in the Fe3+ form. Filtrate was heated to 60 °C, and its pH value was adjusted to 3.2 by adding an appropriate amount of concentrated ammonia. As a result, Fe was separated from tailings and precipitated into Fe(OH)3. Finally, some amount of Fe(OH)3 was washed repeatedly with de-ionized water, and then calcined into Fe2O3. |
2.2 Synthesis of Fe3O4 nano particle
|
Iron hydroxide solve with acid to get FeCl3. Iron sulphate was mixed iron chloride and sodium hydroxide was added drop-wise. Fe3O4 precipitate was aged at 65 degree for 30 min in ultrasonic water bath. To purify prepared Fe3O4 particles, the samples were washed repeatedly with deionized water and ethanol until pH level of was reached. Particles were then dired at 74 degree in vaccum to get Fe3O4. |
2.3 Preparation of colloidal magnetic nanoparticles
|
Dissolved 23.5g ferrous chloride and 8.6 g FeCl2·4H2O in 600ml de-ionized water under N2 with mechanical stirring at 600 rpm (revolution per minute ) and 85°C and then quickly added 30 ml of 7.1M NH4OH. To the resulting suspension 16ml oleic acid was added drop-wise over a period of 30 min. After several minutes, the magnetic precipitate was separated by magnetic decantation and washed with de-ionized water several times, two times with ethanol and finally evaporated to dryness to get iron oxide (Fe3O4) powder. It was further modified with 4 ml of 7.1 M NH4OH to form the hydrophilic magnetic nanoparticles and leading to a stable colloidal dispersion (pH 7) after repeated washing. |
111. EXPERIMENTAL SETUP
|
3.1 Detection mechanism
|
The schematic overview of the detection mechanism of the magnetic scanning imaging method used to detect defects in a ferromagnetic specimen is shown in figure 1.A ferromagnetic specimen is magnetized by a permanent magnet. When there are no discontinuities of physical and chemical characteristics, defects, cracks, and so on, the distortion of magnetic lines of force over the specimen is not much, shown in figure(red circle). When there is a defect, the distortion of magnetic lines of force will be great, shown in figure (red circle). The defect region, considered as the air region, has great reluctance because its permeability is far less than that of the ferromagnetic specimen. Some magnetic lines of force, therefore, bypass the defect region and flow from the vicinity of ferromagnetic material. |
s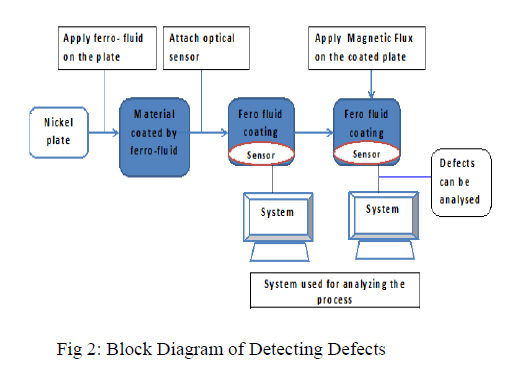 |
In this paper, the magnetization direction of the permanent magnet is perpendicular to the surface of the specimen, along the z-axis, and only the vertical component of the magnetic flux density, Bz, over the specimen is measured: Bz= Bzm + Bz(1) Where Bzm is the vertical component of the incident magnetic flux density produced by the permanent magnet, whose magnitude is almost constant, and Bzis the change of the magnetic flux density generated by the defect and eddy current in the ferromagnetic material: Bz= Bzg − Bze (2) Where Bzg is the change of the magnetic flux density due to the presence of the defect, and Bze is the magnetic flux density produced by the eddy current induced in the ferromagnetic material when a permanent magnet moves over it. Combining a small permanent magnet producing the main magnetic field with the magnetometer measuring the vertical components of the magnetic flux density Bzover the ferromagnetic material, the two-dimensional images of magnetic field fluctuations caused by defects can be obtained by scanning the specimen. |
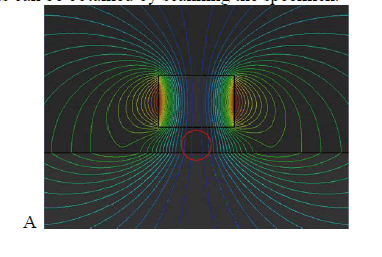 |
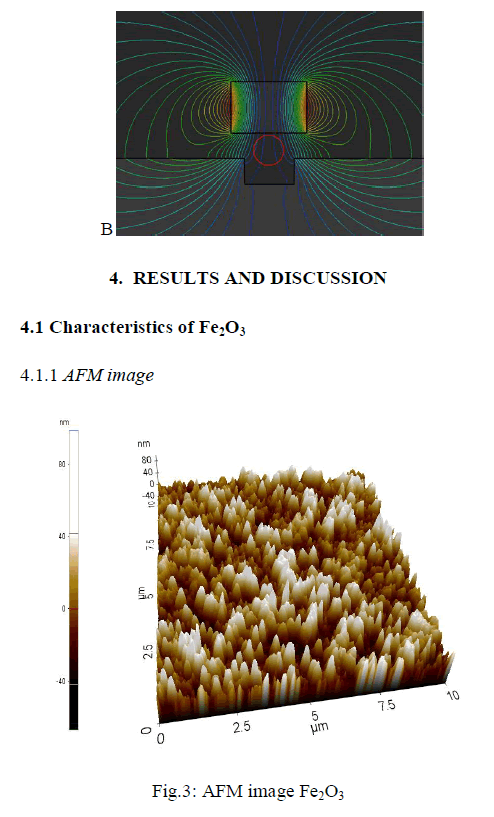 |
The 3D image shows the synthesized iron oxide using coprecipitation method. The topography image obtained from AFM for iron oxide nano-particles increase in particle size for the increase in temperature which ranges from 500 to 800degree Celsius. The particle size is about 60-80 nm. |
4.1.2 Scanning Electron Microscope analysis
|
The SEM analysis is carried out by using Hitachi SUI510, scanning electron microscope. |
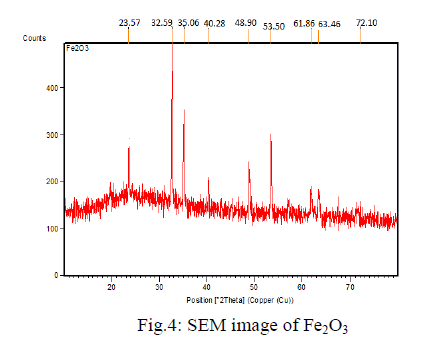 |
The image shows the mono dispersed and uniform size of the particles arranged with spherical shape in nature with a diameter of around 100-200nm. The SEM image shows the particle size and reveals the presence and uniformity of the distributed particles. |
4.1.3 FTIR analysis
|
The FT-IR spectra Fe2O3 is shown in the Fig 4.1.3. The peak value becomes 550cm-1 that corresponds to a strong adsorption band of Fe-O stretching vibrations and the other one at 650 cm-1 region is due to the O-H stretching vibration of the Fe3+-O stretching vibration. |
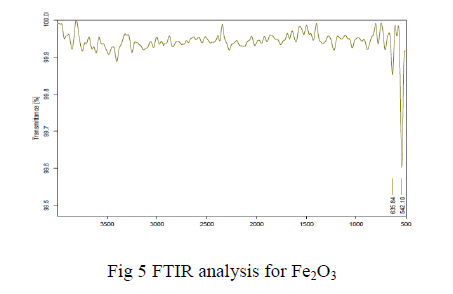 |
4.1.4 X-ray Diffraction analysis:
|
The structural features of Iron oxide are explored from XRD data. The XRD pattern of final powders revealed well developed reflection of Fe2O3 nanoparticle (JCPDS.No. 89- 8104). |
The XRD pattern of the sample exhibits the characteristics peak at 2̬̉ = 24.213o, which corresponds to the (012) plane. It also exhibits peaks at 2̬̉ = 33.255o, 2̬̉ = 35.722o are indexed as planes (104), (110) respectively. The peak at 2̬̉ = 39.408o which corresponds to the (006) plane confirms the presence of Fe2O3 nanoparticle. |
4.2 Characterization of Fe3O4 nanoparticles
|
4.2.1 AFM image:
|
The 3D image shows the synthesized iron oxide using co-precipitation method |
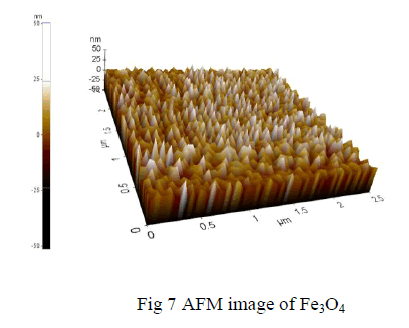 |
The topography image obtained from AFM for iron oxide nano-particles increase in particle size for the increase in temperature which ranges from 500 to 800degree Celsius. The particle size is about 60-80 nm. |
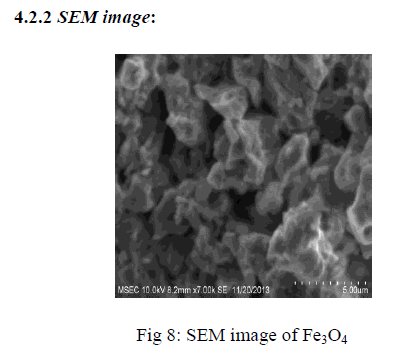 |
SEM image shows the particle size and reveals the presence and uniformity of the distributed particles. It was clear that the particles obtained were in nano size ranging in the diameter from 100-200nm. The SEM image shows the particle size and reveals the presence and uniformity of the distributed particles.. |
4.2.3 FTIR analysis:
|
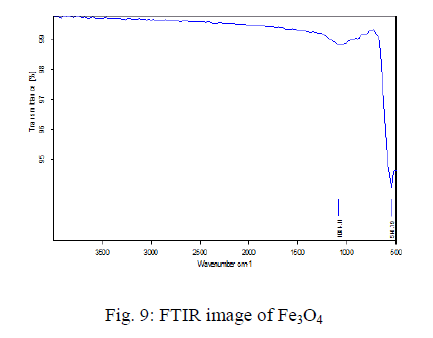 |
The FT-IR spectra Fe3O4 is shown in the Fig 4.2.3. The sample exhibits two strong peaks in the range of 100-510 cm-1 corresponding to the vibrational energies of Fe-O bond. The peak value becomes 550cm-1 that corresponds to a strong adsorption band of Fe-O stretching vibrations and the other one at 650 cm-1 region is due to the O-H stretching vibration of the Fe3+-O stretching vibration.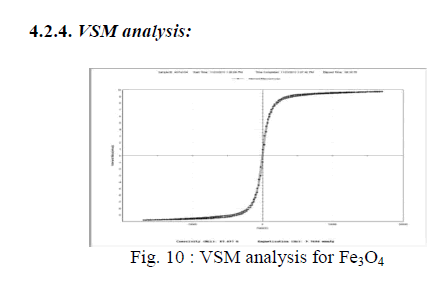 |
Fig: 10 shows the magnetization value is 9.768emul/g and the coercivity value becomes 87.697g. Magnetic properties of Fe3O4 nanoparticles were measured by VSM at 300 K. No magnetic hysteresis loop appears in magnetization curve of Fe3O4 nanoparticles, exhibiting super-paramagnetism. |
4.3 Characterization of colloidal magnetic nanoparticles:
|
4.3.1 SEM image:
|
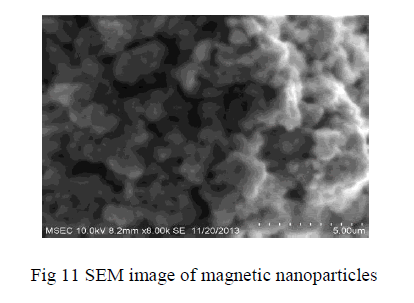 |
SEM image shows the particle size and reveals the presence and uniformity of the distributed particles. SEM image shows the particle size and reveals the presence and uniformity of the distributed particles. It was clear that the particles obtained were in nano size ranging in the diameter from 100-200nm. The SEM image shows the particle size and reveals the presence and uniformity of the distributed particles |
4.3.2 FTIR analysis:
|
The FT-IR spectra colloidal magnetic nanoparticles shown in the Fig12 The sample exhibits two strong peaks in the range of 490-501 cm-1 corresponding to the vibrational energies of Fe-O bond. The FTIR spectra of the iron oxide nanoparticles and micro gel magnetic particles. It was reported that the characteristic absorption band of Fe–O bond of bulk Fe3O4 was at 570 and 375 cm |
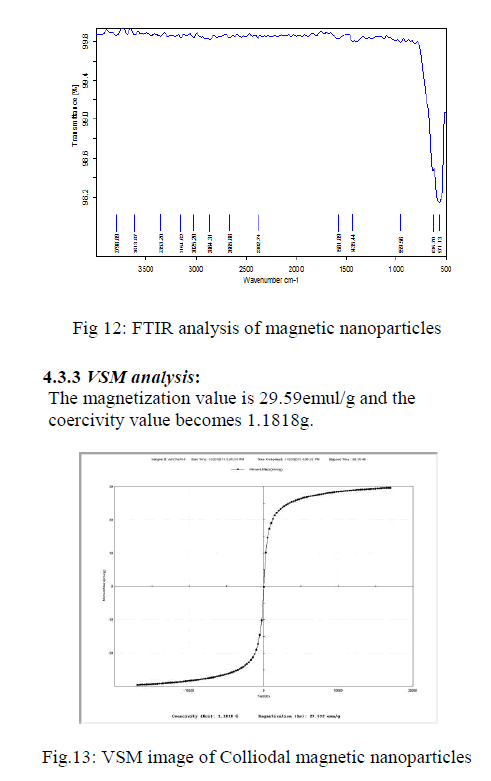 |
It is also without hysteresis, and no sign of finite coercivity field and remnant magnetization can be found from the curve. From the magnetization curve, we can also see that the magnetization does not saturate at 11000 Oe magnetic fields. |
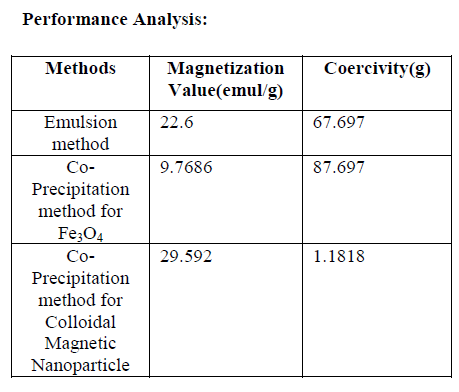 |
`Here the first value of magnetization is higher when compare to other values. But the uniformity good in other two magnetization values. The defects are easily shown by the help of magnetic flux leakage sensor. There are possibilities to further improve the sensitivity by using emulsions with improved stabilization and also with enhanced magnetization fields. |
V.CONCLUSION
|
Nanometer sized Fe3O4 and colloidal magnetic nano particles were prepared by co-precipitation process. The morphological structure and properties of nanoparticles were characterized by using SEM, AFM, VSM, XRD and FTIR analysis to determine the purity and of the particles. Compare to other nanofluids, ferrofluids has good efficiency, magnetization value and the defect can be easily identified in the ferromagnetic materials. The advantage of the nanofluids sensor is that it is simple, in-expensive and covers large area inspection in a short period of time. |
References
|
- V.Mahendran et al (2013), “Naked eyevisualization of defects in ferromagneticmaterials and components” NDT & Einternational-1535
- R.AncyBeautlin et al (2013), “MagneticNanofluid Based approach for imagingdefects”, Journal of nanofluids vol.2, pp, 165-174.
- J.Philip et al (2000), “A new optical techniquefor detection of defects in ferromagneticmaterials and components”, NDT & EInternational 33, 289-295.
- Kikuchi H et al (2011) “Feasibility study ofapplication of MFL to monitoring of wallthinning under reinforcing plates in nuclearpower plants”, IEEE Trans onMagnetics,47,3963-3966.
- Chunyan Xiao et al (2011), “A method ofmagnetic scanning imaging for detectingdefects in ferromagnetic materials”,Measurement Science and Technology, 22,025503(7).
- M.Ravan et al (2010),”Nanofluid based opticalsensor for rapid visual inspection of defects inferromagnetic materials”, IEEE Trans.Magn,46(4),1024.
- Wei Wu-Quanguo He-changzhong Jianget al (2008) “Magnetic iron oxide nanoparticle:synthesis and surface functionalizationstrategies”, Nanoscale Res Lett, 3,397-415.
- T.Nara et al (2007), “A sensor measuring themagnetic flux density for pipe crack detectionusing the Magnetic flux Leakage method”,Appl.Phys.109, 07E305.
- Altschuler E et al (1995), “Nonlinear model offlaw detection in steel pipes by magnetic fluxleakage”, NDT and E International, 28, 1995,35-40
- J.Blitz et al (1991), Electrical and Magneticmethods of non-destructive testing(AdamHilger, Bristol, England)
- BibetteJ et al (1991), “Depletion interactionsand fractionated crystallization for polydisperseemulsion purification”, JournalColloid Interface Science, 147, 474-478.
- Forster F et al (1986), “New findings in thefield of non-destructive magnetic leakage fieldinspection”, NDT Int.19, 3-14.
- Shen Wu et al (2011), “Fe3O4 magneticnanoparticles synthesis from tailings byultrasonic chemical co-precipitation,” MaterialsLetters 65,1882–1884
- Aslam Khan et al (2008), “Preparation andcharacterization of magnetic nanoparticlesembedded in microgels”, Materials Letters62,898–902
- G.A. van Ewijk et al (1999), “Convenientpreparation methods for magnetic colloids”,Magnetism and magnetic materials 201, 31-33.
|