Transportation and industry are two major sectors consuming diesel fuel. Now it has become a challenge for researchers to conserve fossil fuels, which are going to be depleted in near future. In this connection commitment of researchers played a key role in novel design of vehicle. The industry has already improved engine performance significantly with the new technologies and also reduced the pollutant emission levels. More recently, electronically controlled technology is being used in automobile industry as a tool to improve the phase of change. In the advanced technologies of automobile industry the cams are playing a vital role to suit for the ground conditions and fuel consumption. The combustion processes is controlled by operating opening or closing of inlet and exhaust valves. The combustion characteristics are greatly influenced with the fuel preparation process and the distribution of fuel in the engine which is, in turn, controlled by the in-cylinder fluid mechanics. Fuel injection introduces the complexity of describing the physics of vaporizing two-phase flows that vary spatially and temporally from very dense to fairly dilute. Combustion processes and turbulent fuel air mixing are responsible for emission formation. There is a need to have a thorough understanding of these processes to improve performance and reduced emissions without compromising fuel economy. In view of this, an attempt is made to study performance parameters and exhaust emissions for different speed operations.
Keywords |
Conventional engine, LHR, LHR (EEE), Performance and Emissions. |
INTRODUCTION |
Diesel engines are more efficient and known for its functioning in a vital role of both transportation and industrial
sectors. In this connection care to be taken to study the energy conservation phenomenon for effective utilization of
fuel available. It is a known factor that, these engines looses a part of its energy developed during combustion and in
other thermodynamic processes. To minimize these losses, an attempt is made to study a low heat rejection concept as
one of the measures. In LHR engines the effective utilization of generated heat takes place due to insulation of both
piston and cylinder [3-5]. At the same time, the problems associated with high combustion temperatures involved with
LHR engines are resolved. Heavy exhaust blow-down energy and high NOx emissions are two among them, which
leads to decrease in thermal efficiency and inability to achieve legislation levels. |
In this work, an effort is made to minimize the losses by changing inlet cam design mechanism also along with other
measures. The inlet cam design has modified for late closing of intake valve, to overcome blow-down losses [1-2]. In
addition to this, the extended expansion concept has also incorporated to improve the thermal efficiency. |
The performance of the engine is evaluated mainly based on speed and load of the engine. The evaluation may be the
combination of these two or keeping one constant and varying the other. It is to be noted that there is a certain speed,
within this speed range of a particular engine at which the fuel injected per cylinder per cycle will be the maximum [6-
7]. At this point of speed maximum pressure is developed and exerted on the piston. For all practical purposes, the
torque or engine capacity to do work will also be maximum at this point of speed. Hence the importance of speed in the
performance evaluation of the engine is understood and chosen in the present work as the base parameter for evaluating
and comparing conventional, LHR and LHR (EEE) engines. It is also includes the comparison of performance
parameters such as brake power, brake mean effective pressure, brake thermal efficiency, brake specific fuel
consumption and exhaust emissions such as nitric oxide concentration, unburned hydrocarbon, carbon monoxide and
exhaust gas temperature with varying speeds of corresponding engines. |
EXPERIMENTAL SETUP |
An experimental set-up is developed to conduct tests on a four cylinder, four stroke water cooled DI Diesel engine. The
test engine is coupled with eddy current dynamometer. In addition to this, fuel measuring burette, air flow measuring
U-tube manometer are also fitted to the test engine set up. A provision is also made to mount a piezoelectric pressure
transducer flush with the cylinder head surface to measure the cylinder pressure. The experimental set-up layout is
shown in Fig. 1.The equipment and instrumentation used in this work is briefly described below. All paragraphs must
be indented. All paragraphs must be justified, i.e. both left-justified and right-justified. |
PERFORMANCE PARAMETERS |
From Fig.2 - Fig.5 has shown the comparison of Brake Power, Brake Mean effective pressure, Brake thermal efficiency
and Brake specific fuel consumption for conventional engine, LHR engine and LHR (EEE) engine. |
A. Brake Power |
The Fig.2 is shown the trend of Brake power as a function of speed. The Brake power was found to be higher
for LHR (EEE) engine at all speeds than the conventional and LHR Engines. It was observed from the figure that the
Brake power for LHR (EEE) engine is higher about 10% to 13.02% than the conventional engine and LHR (EEE)
engine is higher about 4.10% to 6.73% than the LHR engine. This may be due to higher work done in LHR (EEE)
engine when compared to conventional and LHR engine. |
B. Brake Mean Effective Pressure |
The Fig.3 illustrates the variation of brake mean effective pressure with respect to various speeds for a
conventional, LHR and LHR (EEE) engine. In LHR (EEE) engine the BMEP increases of about 4.10 to 6.73% when
compared to LHR engine and also it is observed that LHR (EEE) engine the BMEP increases of about 10.0% to
13.02% when compared to conventional engine. As the speed increases the BMEP increases and later decreases due to
shorter ignition delay and maximum flame temperature and due to shorter time availability for completion of
combustion as speed increases. |
C. Brake Thermal Efficiency |
The Fig.4 has shown the comparison of simulated and experimental brake thermal efficiency under identical
conditions. The brake thermal efficiency was found to be higher for LHR (EEE) engine at all speeds than the
conventional and LHR engines. This is mainly due to increase in brake power output for the given fuel input than the
conventional and LHR engines. In LHR (EEE) engine maximum of 13.04% and 6.73% when compared to conventional
and LHR engine. |
D. Brake Specific Fuel Consumption |
The Fig.5 has shown the comparison of simulated and experimental brake specific fuel consumption under identical
conditions. It was seen from the graph that the BSFC of the LHR (EEE) engine is found to be decreased from the range
of 10% to 13.02% for all speeds than the conventional engine and it is also observed that the LHR (EEE) engine is
found to be decreased from the range of 4.10% to 6.73% when compared to LHR Engine. This was mainly due to
increase in combustion efficiency so that the fuel consumption can be reduced for the required brake power output. |
EXHAUST EMISSIONS |
From Fig.6 - Fig.9 has shown the comparison of nitric oxide concentration, unburned hydrocarbon, exhaust
gas temperature and carbon monoxide for conventional, LHR and LHR (EEE) engines. |
As very high combustion temperatures are involved with LHR engines resulting in higher NOX formation. The
following discussion is focused on NOX emissions along with other emissions. |
A. Nitric Oxide Concentration |
The Fig.6 illustrates the variation of nitric oxide concentration as a function of speed. It was seen from the
graph that the percentage increase in the nitric oxide concentration in the case of LHR engine are about 34.42% and
7.07% compared to conventional and LHR (EEE) engine. The trend shows that the LHR engines are producing higher
nitric oxide concentration. The increase in nitric oxide concentration in the case of LHR engines is because of the
higher cylinder mean peak temperature. The decrease in nitric oxide concentration in the case of LHR (EEE) engine
when compared to LHR engine is its ability to reduce temperature during the combustion cycle leads to lower
formation rates due to its lower operating temperature because of its lower compression ratio. |
B. Unburned Hydrocarbon |
The Fig.7 has shown the comparison of unburned hydrocarbon as a function of speed. The curve shows that
the UBHC emission for a Conventional engine is higher than LHR and LHR (EEE) engine. Operating temperature of
LHR and LHR (EEE) engine reduce the quenching effect. |
C. Exhaust Gas Temperature |
The Fig.8 has shown the comparison of exhaust gas as a function of speed. The trend shows that the exhaust
gas temperature for conventional engine is lower than LHR and LHR (EEE) engine. LHR engine is reducing the heat
transfer to coolant water. Heat energy carried by exhaust gas is higher in the case of LHR engine. |
D. Carbon Monoxide |
The Fig.9 shows the comparison of carbon monoxide as a function of speed. The curve shows that the CO
emission for a Conventional engine is higher by 4.8% and 16.6% compared to LHR Extended Expansion Engine and
LHR engine. High operating temperature of LHR engine reduces the CO emission. |
CONCLUSION |
• In the comparison of performance parameters and exhaust emissions for conventional engine, LHR engine and
LHR extended expansion engine for all speeds are concluded as below. |
• The Brake power is higher for LHR and LHR Extended Expansion Engine at all speeds than the Conventional. |
• In LHR and LHR Extended Expansion Engine the brake mean effective pressure increases as the speed
increases and later decreases when compared with conventional engine due to shorter ignition delay and
maximum flame temperature and due to shorter time availability for completion of combustion as speed
increases. |
• The brake thermal efficiency is higher for LHR and LHR Extended Expansion Engine at all speeds than the
Conventional engine. This is mainly due to increase in brake power output for the given fuel input than the
conventional engine. |
• Though the trend of the brake specific fuel consumption results are same for all the stages of the engine it was
noted from the experimental results that the brake specific fuel consumption of the LHR and LHR Extended Expansion Engine is decreased for all speeds than the Conventional engine. This was mainly due to increase in
combustion efficiency so that the fuel consumption can be reduced for the required brake power output. |
• The simulated values of the performance parameters discussed above are well comparable with the
experimental values. Simulation is carried out in ‘C’ programming. |
• It observed that the percentage increase in the nitric oxide concentration in the case of LHR engine compared
to Conventional and LHR Extended Expansion Engine. The trend shows that the LHR engines are producing
higher nitric oxide concentration. The increase in nitric oxide concentration in the case of LHR engines is
because of the higher cylinder mean peak temperature. |
• The decrease in nitric oxide concentration in the case of LHR Extended Expansion Engine when compared to
LHR engine is its ability to reduce temperature during the combustion cycle leads to lower formation rates due
to its lower operating temperature because of its lower compression ratio. |
• The UBHC emission for a Conventional engine is higher than LHR and LHR Extended Expansion Engine.
Operating temperature of LHR and LHR Extended Expansion Engine reduce the quenching effect. |
• The exhaust gas temperature for Conventional engine is lower than LHR and LHR Extended Expansion
Engine. LHR and LHR Extended Expansion engine is reducing the heat transfer to coolant water. Heat energy
carried by exhaust gas is higher in the case of LHR engine. |
• The CO emission for a Conventional engine is higher by compared to LHR Extended Expansion Engine and
LHR engine. High operating temperature of LHR engine reduces the CO emission. |
Figures at a glance |
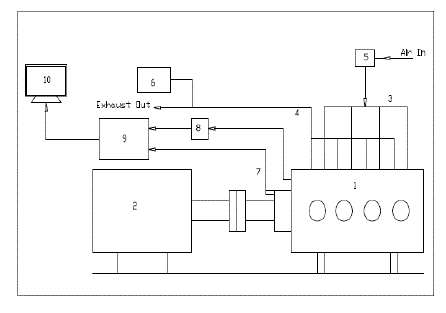 |
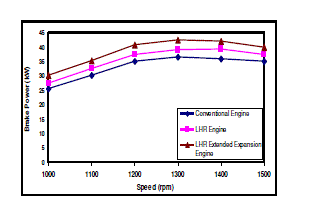 |
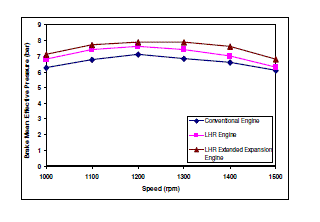 |
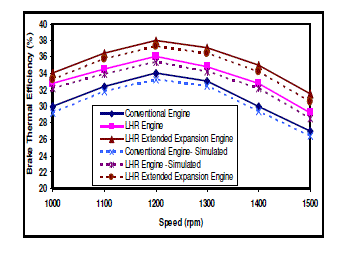 |
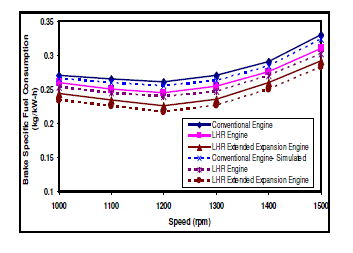 |
Figure 1 |
Figure 2 |
Figure 3 |
Figure 4 |
Figure 5 |
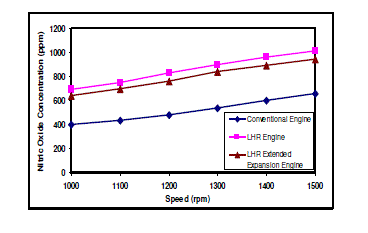 |
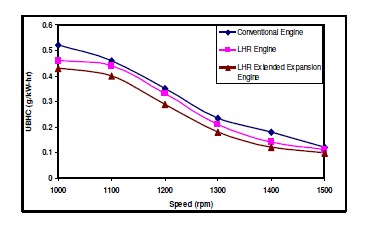 |
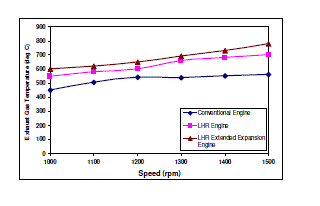 |
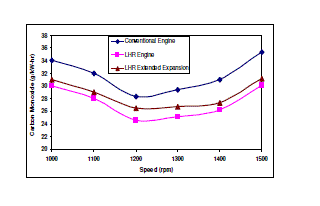 |
Figure 6 |
Figure 7 |
Figure 8 |
Figure 9 |
|
References |
- Tuttle J. H., “Controlling Engine Load by Means of Late Intake Valve Closing“, SAE Paper No: 800794, 1980.
- Tuttle J. H., “Controlling Engine Load by Means of Early Intake Valve Closing“, SAE Paper No: 820408, 1982.
- Jaichandar S. and Tamilporai P., “Low Heat Rejection Engines – An Overview, SAE Paper No: 2003-01-0405, 2002.
- Lavanya N., “Tamilporai P., Chandrasekaran.S and Jancirani.J, Simulation of Expanded and Exhaust Cam in LHR DI DieselEngine,Proceeding of the 19th National Conference on I.C.Engines and Combustion“, Annamalai University, pp: 547-554, 2005.
- Mohd F.Shabir, S. Authars, S. Ganesan, R. Karthik and S Kumar Madhan, “Low Heat Rejection Engines“ – Review, SAE Paper No: 2010-01-1510, 2010.
- Murthy P.V.K, Murali Krishna M.V.S., SitaramaRajuA,Vara Prasad C.M. and Srinivasulu N.V., “Performance Evaluation of Low HeatRejection Diesel Engine with Pure Diesel“, International Journal of Applied Engineering Research, Dindigul Vol:1, No:3, pp: 428-451, 2010.
- B. Rajendra Prasath, P. Tamilporai and Mohd.F. Shabir, Analysis of Combustion, “Performance and Emission Characteristics of Low HeatRejection Engine using Biodiesel“, International Journal of Thermal Science, Vol:49, pp:2483-2490, 2010.
|