Keywords
|
BAN, NM, TN, PN, RN, Channel model, Molecular communication & Nanoscale tools. |
INTRODUCTION
|
Within the last two decades, the outstanding progresses in nano and biotechnology have radically shifted how the human healthcare can be approached. Growing interest in the medical applications of these technologies has recently enabled the emergence of sophisticated nanomedicine applications.For example,a group of bacteria can be genetically engineered to recognize and destroy tumors in the human body . Besides their great potential, the very tiny size and unknown |
physics of therapeutic NMs cause crucial unreliability and uncontrollability problems in these applications. For example, artificial cells used for a smart drug delivery application may be easily exposed to an intrinsic and extrinsic biological noise in gene expression. This makes the drug delivery process completely unreliable and uncontrollable. Through a nanoscale communication paradigm, a network of therapeutic NMs, known as a body area nanonetwork (BAN2), can be realized to enforce reliability and controllability of complex nanomedicine applications. More important, a BAN2 can also coordinate nanomedicine tasks among heterogeneous NM1 populations to reach highly sophisticated behavior and increase the number of design possibilities.For example, a group of non-communicating engineered cells behaves asynchronously and cannot coordinate to perform a predefined task. However, a BAN2 of communicating synthetic cells can overcome the asynchronous behavior problem and coordinate the cell population for a tissue engineering application. Furthermore,a BAN2 may be also required in promising nano medicine applications in order to:- |
(a) Coordinate cooperative NM tasks |
(b) Efficiently gather biosensor data |
(c) Correlate the bio sensory inputs and make Decisions |
(d)Transmit information messages to external entities. |
A BAN2 can also augment various other advancements in the emerging molecular computing systems where logic computations are performed via biological entities. Such computations could also enable fine grained detection of diseases and take action through smart drug release Therefore, a BAN2 can support the communication of NMs needed for such logic computations. In the literature, there are four main nanoscale communication paradigms that may provide the interconnection of the therapeutic NMs in a BAN2. These are nanomechanical, acoustic, electromagnetic, and molecular communication paradigms. In nanomechanical communication, a message is transmitted by a mechanical contact between transmitter and receiver. Acoustic energy such as pressure variation is used for conveying information in acoustic. |
METHODOLOGY
|
As shown in Fig. 1, unlike the early nanomedicine applications, synthetic biology and nano robots-based applications radically Nucleuscel Nucleus cell Relay node Molecular communication of synthetic cells for cell-based smart drug delivery Destination Source Molecular communication of nanorobots for immune system support transform treatment of complex diseases. Inthese applications, through molecular communication, a BAN2 of therapeutic NMs can perform computation and logic operations, and make decisions in the treatment of complex diseases. Here, we review some outstanding examples of these BAN2 proposals.In synthetic biology, molecular communication systems are designed to control the behavior of synthetic cell populations in both time and space. With broadcasting and receiving messenger molecules, molecular communication can enable robust and predictable population dynamics . For example, a molecular communication system is proposed to allow genetically engineered cells to form some predefined spatial pattern. The chemical signal acylhomoserine lactone (AHL) is synthesized and emitted by sender cells to the medium, and receiver cells with band-detect gene networks respond to a threshold AHL concentration by forming a ring-like topology. The given molecular communication system is expected to foster promising nanomedicine applications such as tissue engineering, biomaterial fabrication, and biosensing. Furthermore, in a cell-to-cell molecular communication system with nitricoxide signaling elements is introduced. The given system aims to increase the functionality of synthetic genetic circuits used for coordinating and programming engineered cell populations. It may also serve as a versatile tool in gene therapy applications.Similarly, nanorobot-based nanomedicine applications also requires BAN2s of nanorobots to provide reliable and controllable operations. The collective efforts of the communicating nanorobots can improve the likelihood of successful operations. Molecular communication clearly helps the nanorobots to easily correlate their sensory observations with their close proximity and an external microsystem designed for a health monitoring task . |
MOLECULAR COMMUNICATION MODEL
|
According to the propagation types of carrier molecules, molecular communication can be mainly categorized into two major groups: those with passive transport and with active transport. In passive transport, information is conveyed from a transmitter NM (TN) to a receiver NM (RN) via free diffusion of carrier molecules, as shown in Fig. 3. The TN emits the molecules to the channel, and the emitted molecules freely diffuse in the medium. Some of these molecules electrochemically interact with the receptors of the RN. Such electrochemical contacts provide the reception of transmitted information. In active transport, molecular information is communicated actively using molecular motors (e.g.,kinesin) in molecular rails or microtubules, as shown in Fig. 4. |
MOLECULAR COMMUNICATION WITH PASSIVE TRANSPORT
|
In traditional wireless communication, information messages are encoded via binary bit levels (i.e., bits 0 and 1). These bits also correspond to two different signal levels that are transmitted and received by the transmitter and receiver, respectively. Similarly, a molecular communication channel with passive transport is mostly modeled as a binary channel with two bits, 0 and 1. In the binary channel approach, a single molecule or a number of them are transmitted by a TN at the beginning of the fixedduration time slots to deliver bit 1. If the molecule or a threshold concentration reach the RN within a slot duration, the RN can correctly receive the bit 1. Otherwise, the RN erroneously receives bit 0. In order to deliver 0, the TN transmits no molecule within a slot duration. However, the molecules emitted in the previous slot intervals may reach the RN later when bit 0 is transmitted. This can clearly result in error in the delivery of 0. In order to mitigate such errors, the binary channel approach is also modified by extending the transmission of a single bit to two slot durations. More specifically,00, 01, 10, and 11 are transmitted to deliver bits 0 and 1. Hence, the resultant channel is called a 4-input 2-output channel. In Fig. 5, the communication rates provided by the binary and 4-input 2- output channel approaches are compared for varying values of internode distance between TN and RN. The 4- input 2-output channel approach clearly outperforms the binary channel approach since it mitigates the errors by wisely expanding the Molecule Transmitter nanomachine Receiver nanomachine Molecular communication channel with passive transport Reception of a molecule Receptor Molecular motor Molecular rail Molecular communication channel with active transport Receiver nanomachine Transmitter nanomachine Molecule a Molecule b duration of single bit transmission. For the shorter internode distance values (i.e., 0.1–0.5 cm), the 4-input 2-output channel slightly enhances the rate, while it considerably improves the rate for the relatively longer distances. This result reveals that the 4-input 2-output channel may be more appropriate for nanomedicine applications in which NMs are not relatively close to each other. Apart from the binary and 4-input 2-output channel approaches, passive transport is also analytically modeled by incorporating three different phases of molecular Communication (i.e., molecular emission, diffusion, and reception) into a single channel model. |
MOLECULAR COMMUNICATION WITH ACTIVE TRANSPORT
|
The communication performance of active transport has been characterized mostly by means of an error probability. The molecules, loaded by a TN on molecular motors as shown in Fig. 4, are assumed to degrade and not to reach the RN with error probability pe. If n distinct molecules are used, each of these n molecules is either successfully received with probability (1 – pe) or cannot reach the RN with the probability pe Hence, such a molecular active transport channel can be modeled as an nary erasure channel, and the communication rate of this channel can be given as (1 – pe) log n. In Fig. 6, the communication rate of the n-ary erasure channel is shown with different pe values according to varying numbers of distinct molecules (i.e., n) used in the channel.The rate can clearly be increased with n. By comparing the numerical results in Figs. 5 and 6, it can be concluded that active molecular communication may be more proper than passive molecular communication in terms of providing a higher communication rate. |
However, active molecular communication always necessitates an infrastructure (i.e., molecular rail and motors), while passive molecular communication is not affected by such a restriction. In fact, therapeutic NMs may not require a high communication rate to coordinate and share simple information content in nanomedicine applications. Therefore, active molecular communication may not be preferred if a preset infrastructure is not available. CHANNEL NOISE The characteristics of communication noise in the molecular communication channel also differ significantly from the traditional wireless communication channel. A theoretical model of molecular noise in diffusion-based molecular communication systems is introduced in. |
The effect of noise on molecular communication performance is also investigated by considering various molecular transmission, propagation, encoding, and decoding mechanisms. In addition to these efforts, biological noise , which is inherently observed in inter / intra cellular processes, must be jointly considered foran exact theoretical model of noise. Furthermore, the probability distribution of the noise process is required to be discovered to find the capacity of the molecular communication channel. |
In fact, the noise signal is statistically correlated with the channel input as introduced in .Therefore, the channel input and noise should be jointly considered to investigate the information theoretical characteristics of the molecular communication channel. In addition to this, since the emitted molecules continuously diffuse in the medium until they are received, transient and asymptotic behavior of the noise also need to be investigated. |
RESULTS
|
(a)Deployment&Topology: Therefore, dense deployment of NMs is imperative for nanomedicine applications to reliably convey messages along the highly delicate paths of a BAN2. On the other hand, some nanomedicine applications may require mobile NMs. Unlike the traditional mobility models of ad hoc nodes, in a BAN2, mobility of NMs is mainly governed by extremely different rules such as Brownian motion. For example, in , a communication rate is derived for mobile ad hoc molecular nano networks inspired by a natural immune network consisting of mobile and communicating white blood cells. The molecular communication among NMs is assumed to be provided by means of random collisions of mobile NMs. The analytical evaluations of the rate expression manifest that a BAN2 with mobile NMs can be realized with a sufficiently high end-to-end communication rate. Furthermore, some nanomedicine applications may necessitate a predefined topology of a BAN2. |
For example, a BAN2 may be deployed on some critical points of the human heart for early detection of a possible heart attack. In such an application, all communication parameters must be regulated with respect to the predefined topology to minimize end-to end latency so as to report a possible attack before it occurs. |
DISCUSSION ON FINDINGS
|
(a)Computation,Logic operations and Decisions:In a complex nano medicine applications, a BAN2 of therapeutic NMs performs computation and logic operations, and makes decisions to treat complex diseases. For example, an autonomous biomolecular computer is designed that analyzes the level of messenger RNA and produces a molecule so as to logically control the gene expression processes .However, due to the lack of a central controller,in such applications, all operations must be managed by self-organization of NMs through molecular communication. In these operations, the reliability of molecular communication is crucial in order to efficiently provide computations and logic operations with relatively low error rates. Therefore, reliable and error-tolerant molecular encoding and decoding techniques must be developed for these nanomedicine applications. Furthermore, network information theory may be used to investigate the ultimate computation capability of coordinating NMs in a BAN2. Molecular information flow may also be formulated in order to deduce the computation speed. For example, deterministic molecular information flow in nanonetworks is formulated in, and this formulation can be used to investigate the computational aspects of a BAN2. |
(b)Medium Access Routing: In order to achieve communication performance closer to the information theoretical bounds, efficient networking techniques are also required for the realization of a BAN2. Due to the very low end memory and processing capabilities of self organizing NMs, the developed algorithms entail the minimum level of computational complexity. In , the communication performance of a molecular multiple access channel is investigated. The analytical evaluations reveal that different NMs must access the medium using different types of molecules that can be distinguished at the receiver side. This is because communication bandwidth allocated to each NM reduces to almost zero if the same molecule type is used to access the same channel by a set of NMs . |
Furthermore, the directivity of emitted molecules must be investigated to develop an efficient routing algorithm for the BAN2. In fact, a single molecule may diffuse toward all directions according to the rules of Brownian motion. However,a drift velocity may be used to direct a molecule to a specific direction. Therefore, drift velocity and routes may be jointly considered to develop an efficient routing algorithm. Since the propagation speed of molecules is extremely low, routing algorithms must also be delay-tolerant.For example, mobility of NMs may be exploited to tolerate high delay in such a way that transmission strategies of NMs are regulated according to the mobility patterns of NMs. Such a transmission strategy can reduce the delay. |
CONCLUSION
|
The field of nanomedicine continues to evolve and advance, resulting from extensive research and development efforts in the field of nanotechnology.However, the pace of this development as well as increased opportunities in nanomedicine can be further realized through molecular communication. In this article, we have presented the concept of a BAN2 that exploits molecular communication for the interconnection of NMs. The article discusses current solutions used in nanomedicine, and the nanomedicine applications are further discussed from the perspective of the BAN2. Finally, communication theoretical efforts such as channel capacity, deployment, and topology, as well as networking issues, are reviewed. These issues are further discussed through open research challenges that introduce new interests and development opportunities. Our investigations reveal that the BAN2 with molecular communication is a necessary and promising tool for future nanomedicine in order to provide controllable,reliable, and efficient applications. |
Figures at a glance
|
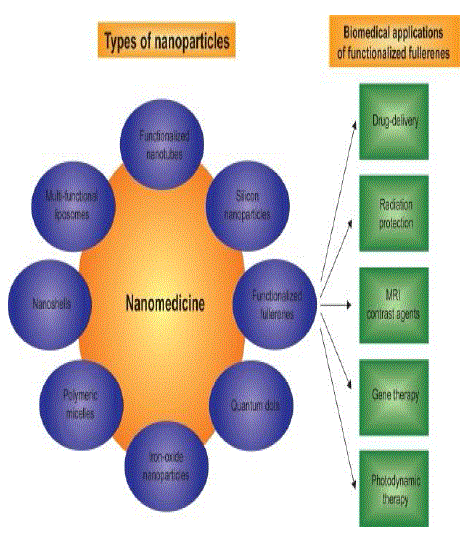 |
 |
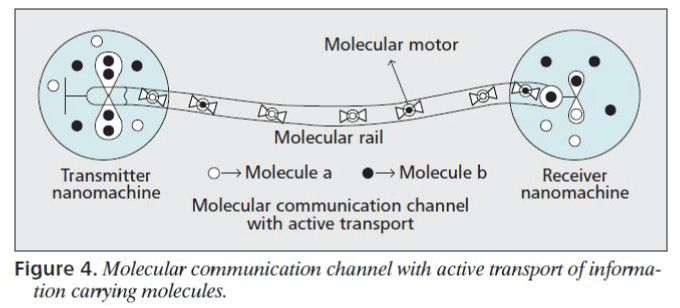 |
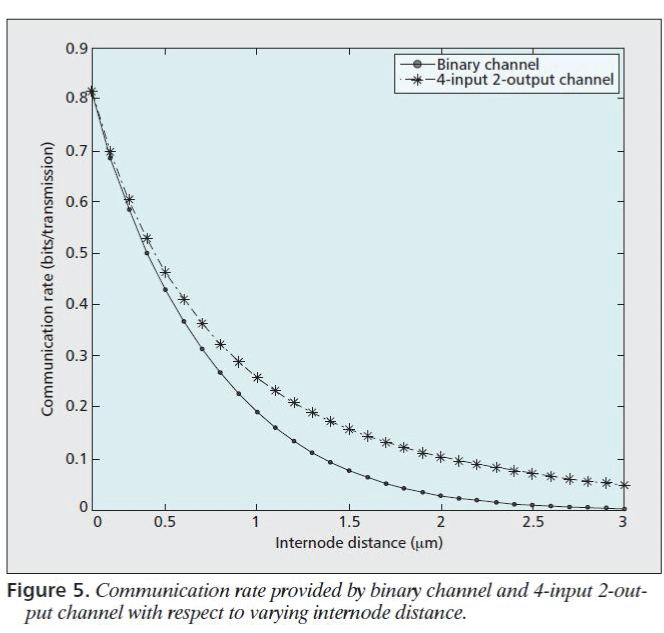 |
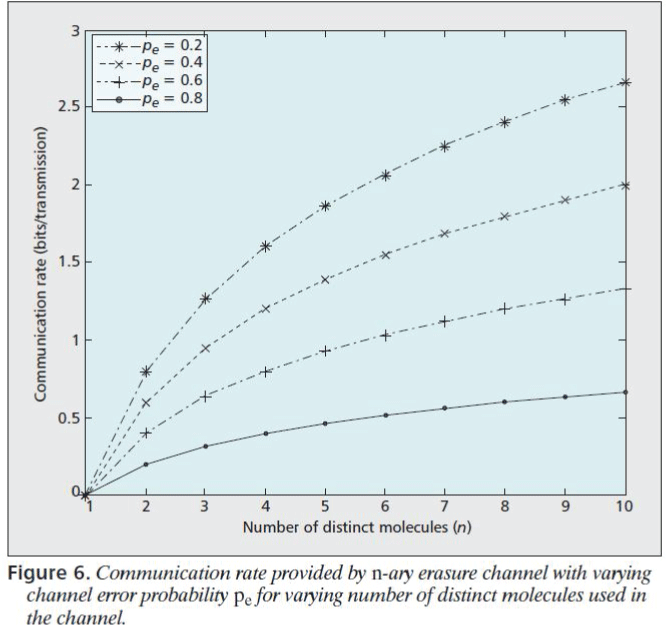 |
Figure 1 |
Figure 2 |
Figure 4 |
Figure 5 |
Figure 6 |
|
References
|
- J. Rodriguez, J.-S. Lai, and F. Z. Peng, âÃâ¬Ãâ¢Multilevel inverters: A survey of topologies, controls, and applicationsâÃâ¬Ãâ. IEEE Trans. Ind. Electron.,vol. 49, no. 4, pp. 724-738, Aug. 2002.
- F. Z. Peng, J. W. McKeever, D. J. Adams, âÃâ¬Ãâ¢A Power Line Conditioner Using Cascade Multilevel Inverters for Distribution Systems,âÃâ¬ÃâConference Record - IEEE Industry Applications Society 32nd Annual Meeting, 1997, pp. 1316-1321.
- L. M. Tolbert, F. Z. Peng, âÃâ¬Ãâ¢Multilevel Converters as a Utility Interface for Renewable Energy Systems,âÃâ¬Ãâ in Proceedings of 2000 IEEE PowerEngineering Society Summer Meeting, pp. 1271-1274.
- G. Sinha, T. A. Lipo, âÃâ¬Ãâ¢A New Modulation Strategy for Improved DC Bus Utilization in Hard and Soft Switched Multilevel Inverters,âÃâ¬Ãâ IECON,1997, pp. 670-675.
- S. Sirisukprasert, J.-S. Lai, and T.-H. Liu, âÃâ¬Ãâ¢Optimum harmonic reduction with a wide range of modulation indexes for multilevel convertersâÃâ¬Ãâ,IEEE Trans. Ind. Electron., vol. 49, no. 4, pp. 875-881, Aug. 2002.
- Xu L, Agelidis VG. âÃâ¬Ãâ¢Active capacitor voltage control of flying capacitor multilevel convertersâÃâ¬Ãâ. IEEE Electr Power Appl 2004
- L. M. Tolbert, F. Z. Peng, âÃâ¬Ãâ¢Multilevel Converters as a Utility Interface for Renewable Energy Systems,âÃâ¬Ãâ in Proceedings of 2000 IEEE PowerEngineering Society Summer Meeting, pp. 1271-1274.
- F. Z. Peng, J. W. McKeever, D. J. Adams, âÃâ¬Ãâ¢Cascade Multilevel Inverters for Utility Applications,âÃâ¬Ãâ Proceedings of 23rd InternationalConference on Industrial Electronics, Control, and Instrumentation, 1997, pp. 437-442.
- Belbaz S, Kadjoudj M, Golea N. âÃâ¬Ãâ¢Analysis of the discontinuous PWM strategies applied to the VSIâÃâ¬Ãâ. In: Proceedings of IEEE intsymponcomputational intelligence and intelligent informatics, Agadir (Morocco); 2007.
- J. S. Lai, F. Z. Peng, âÃâ¬Ãâ¢Multilevel Converters - A New Breed of Power Converters,âÃâ¬Ãâ IEEE Transactions on Industry Applications, vol. 32, no. 3,May 1996, pp. 509-517
- Nandhakumar S, Jeevananthan S. âÃâ¬Ãâ¢Inverted sine carrier pulse width modulation for fundamental fortification in DC–AC convertersâÃâ¬Ãâ. In:Proceedings of IEEE power electronics and drive-systems; 2007. p. 1028–34.
- B. Ozpineci, L. M. Tolbert, and J. N. Chiasson, “Harmonic optimization of multilevel converters using genetic algorithms,âÃâ¬ÃâIEEE PowerElectron. Lett., vol. 3, no. 3, pp. 92-95, Sep. 2005.
- S. Sirisukprasert, J.-S. Lai, and T.-H. Liu, âÃâ¬Ãâ¢Optimum harmonic reduction with a wide range of modulation indexes for multilevel converters”,IEEE Trans. Ind. Electron., vol. 49, no. 4, pp. 875-881, Aug. 2002.
- B. M. Song and J. S. Lai, âÃâ¬Ãâ¢A multilevel soft-switching inverter with inductor coupling,” IEEE Trans. Ind. Application., vol. 37, pp. 628-636,Mar./Apr. 2001.S. M. Metev and V. P. Veiko, Laser Assisted Microtechnology, 2nd ed., R. M. Osgood, Jr., Ed. Berlin, Germany: Springer-Verlag, 1998.
- Y. Liu, Z. Du, A. Q. Huang, and S. Bhattacharya, âÃâ¬Ãâ¢An optimal combination modulation strategy for a seven-level cascade multilevel converterbased STATCOM,âÃâ¬Ãâ in Conf. Rec. IEEE IAS Annual. Meeting, Oct. 2006, —. pp. 1732-1737.
|